Extraordinary Research
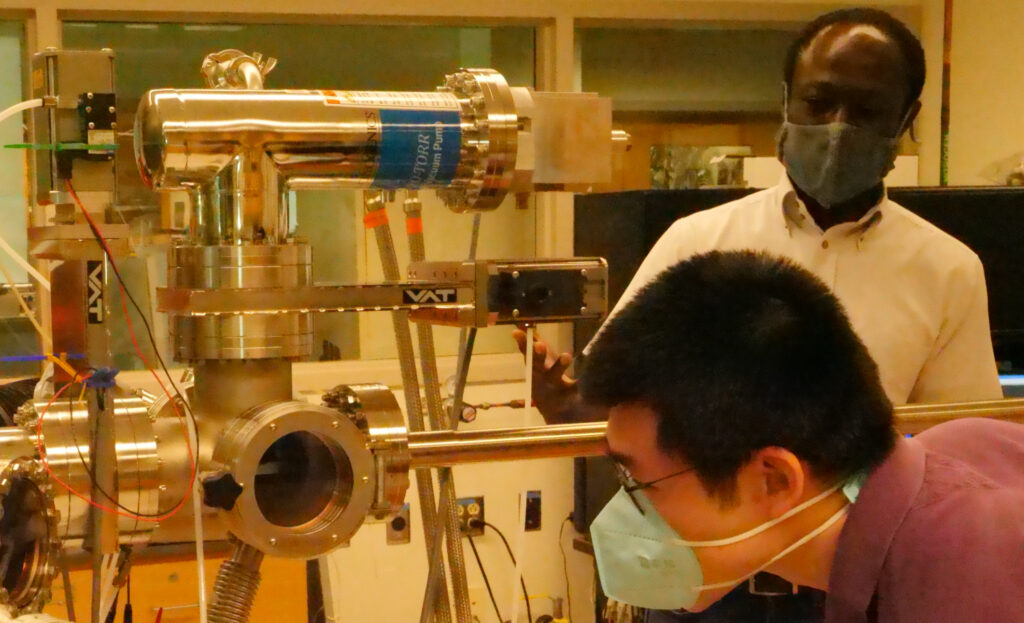
8 AAAS Fellows
Our faculty includes eight fellows of the prestigious American Association for the Advancement of Science.
Our faculty includes eight fellows of the prestigious American Association for the Advancement of Science.